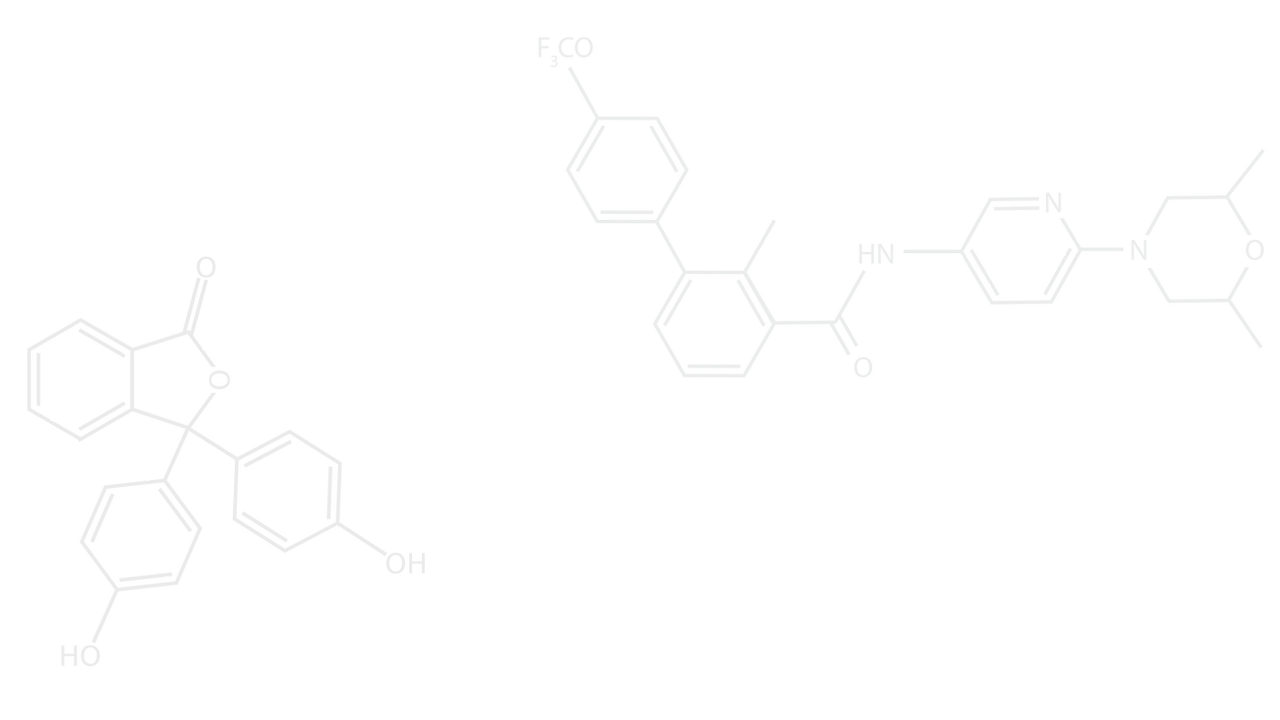


Quantum Light-Matter Dynamics Group

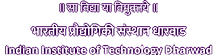
Areas of Interest
​
-
Influences of strong light-matter coupling on the chemical reactivity
-
Reaction mechanisms in molecular physical/chemical processes involving multiple electronic states
-
Multi-reference electronic structure methods and potential energy surfaces
-
Non-adiabatic wave packet dynamics and branching ratios at conical intersections
Strong Light-Matter Coupling
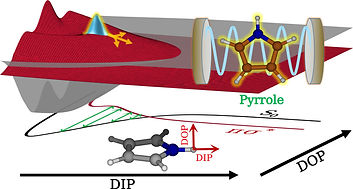
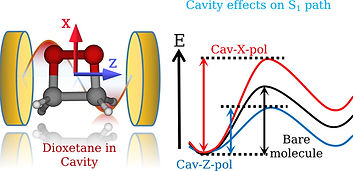
Selectivity is one of the fundamental aspects in chemistry, which involves the steering of a chemical reaction to alter it's final outcome. Achieving the precise selectivity without tedious manipulation of reaction environments has always been a chemist's dream. Recent advancements in cavity quantum electrodynamics and its potential applications to molecular systems have shown that strong light-matter interaction in optical cavities influences the ground and excited state chemical processes. In the presence of an optical cavity, the molecular states can couple to the cavity vacuum field and form light-matter states called as polaritons. These hybrid states manipulate the potential energy surfaces and modify the chemical reactivity. The exploration of polaritonic effects on the molecular processes is advanced recently, although the similar studies on atomic models date back to the 1950s. Furthermore, experimental demonstrations of cavity influences on the chemical processes are under great scrutiny and skepticism, in part, due to the lack of mechanistic understanding. Therefore, theoretical treatments of such systems may play an important role in providing the validity of experimental studies and establishing the general principles of polaritonic chemistry. We are interested in exploring the mechanistic details of cavity modified chemistry in molecular systems and develop computationally accessible tools to investigate the underlying principles of polaritonic phenomenon. The obtained insights would assist in offering the novel pathways for the control of chemical reactivity and provide new applications.
Photo-Chemistry
Photo-chemistry entails the interaction of light with matter and plays a prominent role in different areas of science such as synthetic chemistry, biology, and materials science. The irradiation of UV light results the molecule to get electronically excited and the corresponding reaction dynamics differs significantly from the ground-state chemistry. The ground-state reactions are generally controlled by the transition state (first-order saddle point) points along the minimum energy path toward the product. Whereas the excited state molecular transformations are largely governed by the crossings of potential energy surfaces called as "conical intersections (CIs)". In the vicinity of CIs, the electronic and nuclear degrees of freedom become strongly coupled causing the breakdown of Born-Oppenheimer approximation. The complex non-adiabatic phenomenon, originating from CIs, consequently governs the final outcome of a photochemical reaction. In order to describe the non-adiabatic phenomenon adequately, multi-reference electronic structure methods along with quantum dynamics are essential. Due to the intrinsic complexity of the multi-reference electronic structure methods, advanced knowledge of quantum chemistry is required to employ them. We aim to understand the in-depth mechanistic details of complex photochemical processes such as photosynthesis, DNA damage, and photo-catalysis.
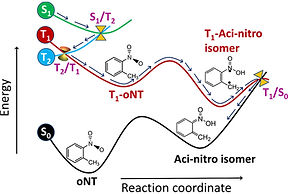
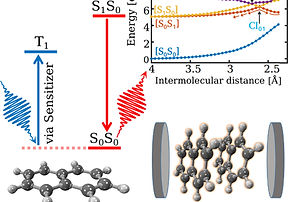
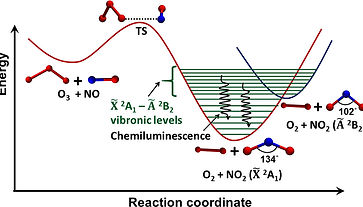
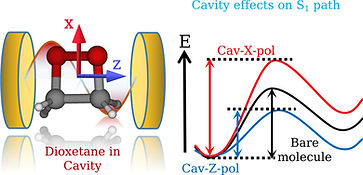
Chemiluminescence
Chemiluminescence process has also been found to be involving conical intersections and corresponding non-adiabatic regions of potential energy surfaces. This phenomenon however generates light during a thermally activated chemical reaction and is quite opposite to the photoinduced chemical reaction. We would have witnessed this fascinating Glow-in-the-Dark phenomenon already, either as a kid mesmerized by the glowing fireflies at night or as a curious chemist wondering about the emission of intense blue light in luminol chemilimunescence reaction. The reaction mechanism of chemiluminescence has been extensively investigated by several theoreticians and experimentalists with different molecular models representing firefly dioxetane. However, the mechanistic studies on other class of chemiluminescence reactions are quite limited and general mechanistic principles of the chemiluminescence process are still need to be obtained. Our interest lies in the theoretical exploration of gas-phase chemiluminescence systems to gain the fundamental level understanding of the reaction mechanism.